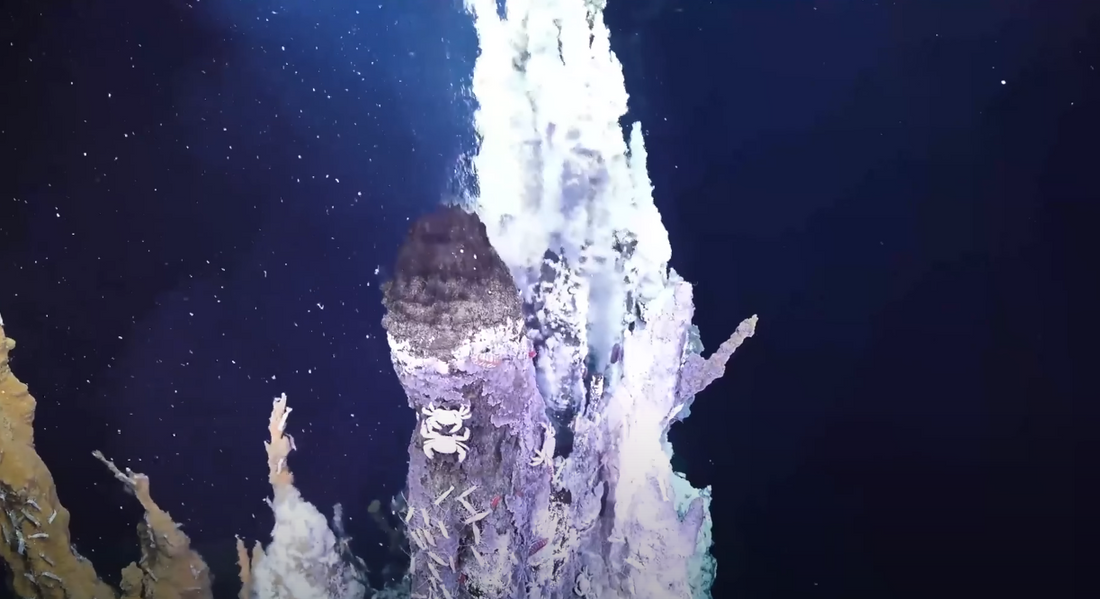
Synthetic Aperture Radar (SAR) Doppler Tomography in Marine Environments: Potential and Challenges
Share
The Challenges of SAR Doppler Tomography in Marine Applications
While SAR Doppler Tomography presents emerging possibilities in marine applications, it is crucial to acknowledge its current limitations before assuming its widespread utility. One of the primary challenges is its depth penetration in marine environments. Unlike sonar systems that use acoustic waves, which travel efficiently through water, SAR operates on electromagnetic waves, which experience rapid attenuation in conductive saltwater. As a result, SAR penetration into the seafloor is highly constrained. Studies suggest that while lower-frequency SAR, such as P-band (0.3-1 GHz), can penetrate up to 30 meters in dry sediments, this capability is drastically reduced in saltwater-dominated environments where penetration depth is often limited to just a few meters or less (Ulaby et al., 2014).
Furthermore, interpreting SAR data in marine conditions is complicated by environmental noise, including wave motion, variable salinity, and temperature gradients. These factors can distort radar reflections, making it challenging to accurately reconstruct subsurface images. The resolution of SAR at lower frequencies is also a limiting factor; while it may detect larger anomalies such as buried wreckage or geological formations, it is unlikely to provide the fine detail needed for precise subsea mapping. Additionally, SAR Doppler Tomography requires multiple passes and varying angles to reconstruct 3D images, which may not be feasible in dynamic oceanic conditions (Raney, 1998).
Another challenge is the misconception that SAR can fully replace existing subsea exploration tools like sonar and ground-penetrating radar (GPR). Sonar systems remain superior for deep-sea mapping due to their ability to propagate through water without significant attenuation. Similarly, GPR is better suited for shallow water and coastal applications where electromagnetic waves can still achieve reasonable penetration in specific substrates. While SAR Doppler Tomography can complement these technologies by providing large-scale, remote sensing capabilities, its role should be carefully evaluated to avoid overstating its effectiveness for deep-sea exploration (Mast et al., 2020).
How Deep Can SAR Penetrate?
The penetration depth of Synthetic Aperture Radar (SAR) depends on the wavelength (frequency), material properties, and moisture content of the surface being imaged. In dry conditions, low-frequency SAR can penetrate several meters to tens of meters.
Typical SAR Penetration Depths
Frequency Band | Wavelength | Penetration Depth (in Dry Soil/Rock) | Common Uses |
---|---|---|---|
X-band (8-12 GHz) | ~3 cm | Negligible (millimeters) | High-res surface imaging (e.g., terrain mapping) |
C-band (4-8 GHz) | ~5 cm | A few centimeters | Surface monitoring (e.g., Sentinel-1) |
L-band (1-2 GHz) | ~23 cm | Up to 10 meters | Vegetation, ice, and shallow ground penetration |
P-band (0.3-1 GHz) | ~1 meter | Up to 30 meters (in dry conditions) | Deep soil/rock penetration, archaeology, and forestry |
VHF/UHF (Below 300 MHz) | Several meters | Up to 100 meters in dry sand/ice | Subsurface imaging, military, geology |
Potential Applications and Future Developments
Despite these limitations, SAR Doppler Tomography remains an emerging technology with potential applications in specific marine environments. It has shown promise in detecting buried objects beneath the seafloor in shallow, sediment-rich areas, where penetration depths of up to a few meters could be useful for archaeological and geological studies. Additionally, SAR has been successfully used in ice-covered environments, where it can penetrate deeply into glaciers and ice sheets, revealing subglacial features that would be otherwise inaccessible (Rignot et al., 2011).
One area where SAR Doppler Tomography could contribute is in detecting methane seeps and hydrothermal vents. These geological features play critical roles in marine ecosystems and climate regulation but are difficult to locate using traditional sonar alone. By analyzing Doppler shifts in SAR data, researchers may be able to infer subtle seafloor disturbances indicative of gas emissions or volcanic activity beneath the ocean floor (Cazenave et al., 2016). However, further research is required to refine this approach and validate its effectiveness under real-world oceanic conditions.
Future advancements in SAR technology may also help address current limitations. The integration of SAR with other remote sensing methods, such as multi-beam sonar, LiDAR, and autonomous underwater vehicles (AUVs), could provide a more comprehensive approach to marine exploration. Additionally, advances in signal processing and machine learning may improve the interpretation of SAR data, allowing for better differentiation between noise and meaningful subsurface reflections. The development of hybrid SAR systems optimized for subsea imaging could also expand our ability to map the ocean floor with improved resolution and depth penetration (Carrara et al., 2004).
As interest in maritime security, offshore resource management, and oceanographic research continues to grow, SAR Doppler Tomography presents an innovative but still developing tool for deep-sea sensing. While it is unlikely to replace existing technologies in deep-sea exploration, it has potential as a supplementary method for broad-area surveys, particularly in shallow water and ice-covered environments. By continuing to refine SAR’s capabilities and integrating it with complementary sensing technologies, researchers may unlock new insights into the hidden landscapes beneath our oceans.
References
-
Carrara, W. G., Goodman, R. S., & Majewski, R. M. (2004). Spotlight synthetic aperture radar: Signal processing algorithms. Artech House.
-
Cazenave, A., Biancale, R., & Lemoine, F. (2016). Satellite radar altimetry: Observing changes in Earth's environment. Cambridge University Press.
-
Mast, J. E., Johansson, E. M., & Alqadah, M. (2020). Remote sensing using low-frequency synthetic aperture radar in archaeological and geological applications. IEEE Geoscience and Remote Sensing.
-
Raney, R. K. (1998). Radar fundamentals: Technical perspective for remote sensing. IEEE Transactions on Geoscience and Remote Sensing.
-
Rignot, E., Mouginot, J., & Scheuchl, B. (2011). Ice flow of the Antarctic Ice Sheet. Science, 333(6048), 1427-1430.
-
Ulaby, F. T., Moore, R. K., & Fung, A. K. (2014). Microwave remote sensing: Active and passive. Artech House.